Human hibernation: Secrets behind the big sleep
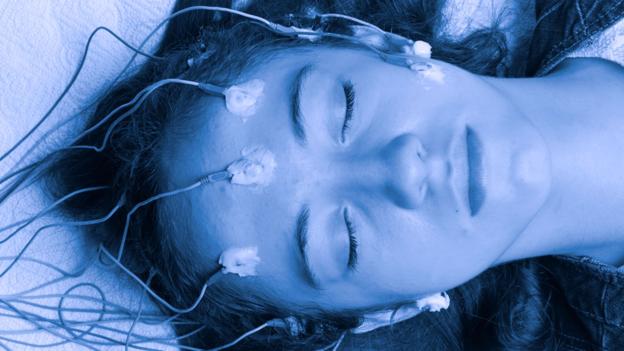
Human hibernation: Secrets behind the big sleep (Thinkstock)
Imagine it: you have been rushed into the emergency room and you are dying. Your injuries are too severe for the surgeons to repair in time. Your blood haemorrhages unseen from ruptured vessels. The loss of blood is starving your organs of vital nutrients and oxygen. You are entering cardiac arrest.
But this is not the end. A decision is made: tubes are connected, machines whir into life, pumps shuffle back and forth. Ice-cold fluid flows through your veins, chilling them. Eventually, your heart stops beating, your lungs no longer draw breath. Your frigid body remains there, balanced on the knife-edge of life and death, neither fully one nor the other, as if frozen in time.
The surgeons continue their work, clamping, suturing, repairing. Then the pumps stir into life, coursing warm blood back into your body. You will be resuscitated. And, if all goes well, you will live.
Suspended animation, the ability to set a person’s biological processes on hold, has long been a staple of science fiction. Interest in the field blossomed in the 1950s as a direct consequence of the space race. Nasa poured money into biological research to see if humans might be placed in a state of artificial preservation. In this state, it was hoped, astronauts could be protected from the dangerous cosmic rays zapping through space. Sleeping your way to the stars also meant carrying far less food, water and oxygen, making the ultimate long-haul flight more practical.
There has long been interest in whether suspended animation could allow astronauts to survive missions to Mars and beyond (Science Photo Library).
One recipient of that funding was a young James Lovelock. The scientist would dunk hamsters into ice baths until their bodies froze. Once he could no longer detect a heartbeat, he would reanimate them by placing a hot teaspoon against their chest (in later experiments, Lovelock warmed to the space-age theme by building a microwave gun out of spare radio parts to more gently revive his test subjects). These experiments on the flexibility of life would set him on the path to his most famous work, the “Gaia hypothesis” of the world as a living super-organism.
Adventurous as they were, these early experiments did not progress beyond the animal stage, and astronauts were never frozen and revived with hot spoons. The idea of transforming people into inanimate bars of flesh for long-distance space travel remained in the realm of science fiction. Nasa’s interest tailed off with the end of the space race, but the seeds planted by Lovelock and his colleagues continued to grow.
Cold storage
In 1900, the British Medical Journal published an account of Russian peasants who, the author claimed, were able to hibernate. Existing in a state approaching “chronic famine”, residents of the north-eastern Pskov region would retreat indoors at the first sign of snow, and there gather around the stove and fall into a deep slumber they called “lotska”. Waking once a day to wash some hard bread down with water, the family took it in turns to watch the fire, only rousing themselves fully once spring had broken. No trace of the sleepy peasants of Pskov has ever emerged since, but the fantasy of human hibernation persists, and very occasionally, something that looks very similar to it crosses into reality.
A century later, Anna Bagenholm was on a skiing holiday in Norway when she crashed head first into a frozen stream and became trapped under the ice. When rescuers finally arrived, the Swedish radiologist had been submerged for 80 minutes, and her heart and breathing had stopped. Doctors at Tromso University Hospital recorded a body temperature of 13.7C, the lowest ever observed in a victim of accidental hypothermia. By all accounts she appeared to have drowned. And yet, after careful rewarming and ten days spent in intensive care, Bagenholm woke up. She went on to recover almost fully from her cold brush with death. Under normal circumstances, even a few minutes trapped underwater would be enough to drown a person, and yet Bagenholm had survived for over an hour. Somehow the cold had preserved her.
It’s not the first time the benefits of cold for traumatic injury have been made apparent. As far back as the Napoleonic era, medics noted that wounded infantrymen left out in the cold had better survival rates than the wounded officers kept close to the fire in warmed tents. Therapeutic hypothermia is now commonly used in hospitals to reduce injury in a wide variety of situations, from surgery to helping infants recuperate following difficult births.
Lowering your body temperature slows your metabolic activity, about 5–7% for every degree dropped. This in turn reduces the rate at which you consume essential nutrients such as oxygen. Tissues that might become starved of oxygen due to blood loss or cardiac arrest are thus protected. In theory, if we were to keep reducing your temperature, eventually your biological processes would come to a standstill. You would exist in a state of suspended animation. Like a stopped clock, there’d be nothing physically wrong with you – all the components inside would still be intact, simply stationary. All it would take would be a little heat to set you in motion again.
Of course, it’s not that simple. Hypothermia is dangerous. Your body wants to be warm and will fight to remain that way. Throughout your life, it will maintain a fairly constant temperature of around 37C. This requires great effort. Your body must perform countless constant adjustments to balance heat production with heat lost to the environment, working to keep your temperature within a narrow band. If it drops too low, your blood is shunted away from the exposed skin and gathers in your central torso while you shiver and huddle under blankets. The effects of more severe cold are disastrous. At a body temperature of around 33C – just four degrees below normal – your heartbeat begins to flutter. At 25C, there’s a risk it will stop altogether. And even if you survive hypothermia, warming you up again can cause extensive kidney damage.
Arctic ground squirrels make sure their bodily fluids don’t freeze solid during hibernation (Science Photo Library)
There are, however, certain species of animal that can endure far greater spells of cold. The Arctic ground squirrel normally maintains a body temperature similar to our own. But during hibernation, it can survive a core temperature as low as –3C, carefully managing its super-cooled bodily fluids so that it won’t freeze solid. And Lovelock’s hamsters could survive hypothermic depths that would kill us. How animals survive these states is of great interest to anyone hoping to unlock the secrets of suspended animation for humans.
Staying alive
“When is your comrade dead?” asks Professor Rob Henning with a grin, quoting an Army handbook he received as one of the Netherlands’ last draft of conscripts. “One: Is he rotting? Two: Is his head more than twenty centimetres from his body?” Like Lovelock, Henning has conducted experiments with hibernators that have given him a flexible view of what constitutes being alive.
From the top floor of the Department of Clinical Pharmacy and Pharmacology at the University Medical Centre Groningen (UMCG), a large window looks down on the medieval city spread over a pancake-flat landscape. Below is a bustling hospital, the region’s hub for transplant surgery. It’s also where Henning and his team are uncovering the secrets of hibernation.
“What we’re doing here is biomimicry,” says Henning, “using these great adaptations in nature to hijack them for the benefit of medicine.”
Many animals can slow their metabolism to enter low-energy states: insects, amphibians, mammals, birds and fish. In short periods, this condition – characterised by reduced body temperature and inactivity – is known as torpor. By stringing together many of these short sessions of torpor, animals can enter the long-term dormancy we call hibernation. With this technique, small animals such as mice, hamsters and bats can last out the cold famines of winter huddled away, conserving energy.
Trained as an anaesthetist, Henning started “hobbying” in hibernation in the 1990s, but things took off in earnest when his research group was formed around six years ago. “If you think about hibernators, you have a lot of applications. The most obvious ones are any type of major surgery,” he explains. Blood loss is the major cause of death during surgery, but in their hypothermic state, hibernators can survive far worse injuries than they can at normal body temperatures. This is partly because tissues are protected at low metabolic rates, and partly because the heart is pumping blood at a fraction of the rate it usually does.
A dormouse in torpor. It will spend up to three quarters of its life asleep, hibernating (Science Photo Library)
But a resistance to cold and blood loss isn’t the total sum of hibernators’ incredible endurance. Although it resembles a very long lie-in, hibernating is not a simple matter of sleeping through the cold. It’s a gruelling marathon of hypothermia, starvation and disease susceptibility. To endure these sufferings, the animals that practice it have developed a suite of adaptations to protect mind and body.
Before a long hibernation, animals eat their way into obesity, essentially becoming type 2 diabetic. Unlike in humans, this does not result in the thickening of artery walls that leads to heart disease. Some species will stop eating two or three weeks before hibernation, suddenly resistant to the pangs of hunger even while maintaining their regular level of activity.
While a human can lie in bed for a week before muscles begin to atrophy and blood clots form, hibernators will endure months without moving. During hibernation, the microbiome – the community of bacteria living in an animal’s digestive tract – is battered by cold and the sudden lack of food. Hibernators’ lungs become covered with a thick deposit of mucus and collagen like those seen in people with asthma, and their brains show changes that resemble those of early-stage Alzheimer’s. Some species lose memory during hibernation. Most surprising of all, some show symptoms of sleep deprivation when they finally wake. And yet, hibernators are able to counter all of these issues to bounce back in spring, often without any long-term ill effects.
Thicker than water
UMCG is a half-kilometre complex of buildings so tightly huddled together that it’s possible to walk from the grand foyer at one end to the bicycle racks at the other without stepping outside. One of these buildings is the animal laboratory.
In a tiny room set away from the main corridor, Henning’s doctoral student Edwin de Vrij and his colleague are tending to a rat laid prone on a bed of ice. A tangle of fine tubes and wires surrounds the animal, delivering life-preserving fluids and carrying away precious data. A spool of paper inching from one machine shows that from a frenetic 300 beats per minute, the rat’s heart rate has slowed to just 60. The red numbers glowing on another show that the rat’s internal temperature has dropped more than 20 degrees to 15C. Clicking like a metronome, a ventilator delivers steady breaths to the anaesthetised rodent. As a non-hibernator like us, the rat cannot survive deep hypothermia without medical assistance. “If you cool them down, nerve impulses will be slower, and muscles have a harder time in the cold, so it’s quite physiological that they have a harder time breathing,” explains de Vrij. This isn’t the case for true hibernators – or some other non-hibernating mammals, for that matter. “Somehow hamsters can maintain adequate breathing,” he says. “We don’t have to ventilate them.”
As well as inducing hibernation in hamsters (a process that takes weeks of gradual adjustment in climate-controlled rooms to mimic the onset of winter), the UMCG team also induce forced hypothermia states like that of our rat, chilling the animals rapidly until they fall into a state of metabolic suspension.
Today, de Vrij is searching for platelets, which are essential for blood clotting to prevent bleeding. Hibernating animals avoid getting blood clots despite their lack of activity, an ability that comes down partly to a curious change in the hypothermic body: as they cool, platelets disappear from the blood. Nobody yet knows where they go, but their prompt reappearance on rewarming has de Vrij convinced that they are preserved somewhere in the body, rather than being absorbed and later resynthesised. Surprisingly, this change also happens even in non-hibernators, including rats and – occasionally – human victims of hypothermia.
Platetets (pink) are vital for blood clotting, and could play a key role in helping animals survive during hibernation (Science Photo Library)
The shared characteristics of different hibernators mean it’s likely that these species have inherited fragments of protective mechanisms against cold, inactivity, starvation and asphyxiation from common ancestors and developed these into a comprehensive low-metabolic syndrome. There are even hints that we humans might, to some extent, retain some of these abilities. For a long time, there was no evidence that primates could hibernate. But in 2004, a species of Madagascan lemur was shown to practice regular bouts of torpor. “If you look at the lemur and look at us, we share about 98% of our genes,” says Henning. “It would be very strange if the tools of hibernation were all packed into that 2% difference.”
As their body temperature drops, hibernators also remove the lymphocytes (white blood cells) from their blood and store them in the lymph nodes. And within 90 minutes of awakening, these reappear. This damping down of the immune system prevents a general inflammation in the body during rewarming – the very thing that would cause humans and other non-hibernators to suffer kidney damage. However, it’s a risky strategy, leaving animals unable to mount an immune defence while hibernating. The fungus responsible for white-nose syndrome, currently wiping out bat colonies in the USA, takes advantage of this vulnerability, infecting the bats while they are dormant. In response, the bats frequently exit hibernation and rewarm to fight off the pathogen – the high-energy cost of these interruptions ultimately killing them.
Funny smell
Knowing how hibernators control these changes in their blood could have immediate and far-reaching benefits for us. As well as improving our ability to survive hypothermia and cold suspended-animation states, stripping the blood of white blood cells could prevent the aseptic sepsis caused by heart–lung machines, in which activation of blood cells as they pass through the life-support equipment triggers a body-wide immunological reaction. Transplant organs, often chilled for transport, would also benefit from better cryoprotection. And we could increase the shelf-life of our blood stocks – we still haven’t figured out how to store donated blood platelets at low temperatures, so blood donations can only be kept a week before they must be used or thrown away due to the risk of bacterial infection.
The UMCG team took a giant leap towards achieving these goals quite by accident after a student left a culture of hamster cells in a fridge at 5C. After a week the hamster cells were still alive, and smelling of rotten eggs. The student poured the medium surrounding the cells over a separate batch of cells from a rat, suspecting the smelly cells might have secreted some kind of protective agent. She placed them in the same fridge and waited. Normally, refrigerating rat cells would quickly kill them, but after two days they were still alive.
The team is investigating several compounds that might be responsible for this cryopreservation. One is an enzyme known as cystathionine beta synthase (CBS), which stimulates the production of hydrogen sulphide, the molecule that gives rotten eggs their characteristic whiff. If hamsters are injected with a chemical to inhibit CBS, they can no longer enter torpor, and those that were forced into hypothermic states suffered the kind of kidney damage one would expect in non-hibernators like us.
Of over a hundred compounds Henning’s team has investigated, many had no effect, but a few did, conferring long-term cold protection to cell samples. The team has already patented one of these compounds, Rokepie, as an additive. This would allow cells that normally need to be kept at 37C, such as those from humans or mice, to be stored in the refrigerator, either for transport or so experiments can be put on hold during weekends and busy periods.
The leading cryopreservation molecules extracted from hibernators are incredibly potent, and it seems they work by eliciting changes in the cells themselves – whether these are from hibernators or not. If so, this offers further evidence that we still possess some tools that could help endure hypothermia and low metabolic states.
For now, applying the lessons they’ve learned from hibernators wholesale onto humans is not within the remit of Henning’s group. The space race is long over, and Nasa is not awarding major grants to develop suspended animation. However, the US Army is.
Golden hour
“If you look anywhere near a trauma bay, things are pretty chaotic,” says Professor Sam Tisherman. “It’s controlled chaos, but the chaos mainly comes from the fact you never know what’s going on with the patient.”
In frenetic hospital emergency wards, it’s often not possible for doctors to identify the problem, fix it and keep the patient alive all at the same time. Patients suffering uncontrolled blood loss, for example, may go into cardiac arrest. When this happens, surgeons must fight the clock to stop the bleeding before they can start resuscitation efforts. “Somebody rolls in and they’re basically dying,” says Tisherman. “We’re quickly trying to resuscitate them, and figure out what’s wrong with them, and repair injuries all at the same time.” This is the fundamental underpinning of trauma medicine: you are always against the clock.
Tisherman wants to buy doctors a little more time. He believes that by inducing hypothermia we can extend the “golden hour” in which surgeons battle to save the lives of critically injured patients. To do this, he’s pushing human endurance of hypothermia far beyond its normal limits.
After graduating from MIT in 1981, Tisherman built a career in critical care medicine. He won a Lifetime Achievement Award in Trauma Resuscitation Science from the American Heart Association in 2009, and is now an Associate Director of the Safar Center for Resuscitation Research in Pittsburgh. It was founded by Peter Safar, the Austrian physician who popularised the “kiss of life”, CPR, and drove the creation of the Resusci Anne doll used in teaching it. At Pittsburgh, Safar created the world’s first intensive care training programme. His lifelong aim was to “save the hearts and brains of those too young to die.”
The procedure that Tisherman is pioneering is called emergency preservation and resuscitation. His work is supported through the US Army’s Telemedicine and Advanced Technology Research Center, which funds research on topics as niche as advanced prosthetics and robots to carry wounded soldiers out of the battlefield.
Some of his surgeons will already be familiar with hypothermic techniques, having routinely chilled patients to the low 30s or high 20s. For procedures that require zero blood flow, cardiac surgeons will even cool patients to around 15C, the point at which their heart stops.
Surgeons performing a cardiac operation using hypothermic protection of the patient in Russia in 1979 (Science Photo Library)
Tisherman is planning to cool patients to this point, and perhaps even further, chilling them to such a degree that the entire body enters a kind of suspended animation. During this time, they will have no heartbeat, no breathing and no discernible brain activity. In fact, they’ll have no blood, either – it will be drained and replaced with ice-cold saline, the only way to cool a human fast enough to avoid tissues becoming damaged as they struggle to remain functioning. Tisherman calls this state “hypothermic preservation”.
The procedure has already been demonstrated successfully in the lab, reviving dogs that had lain suspended in cold states for up to three hours. Trials are now moving to a clinical setting. Surgeons, anaesthetists and perfusionists at Massachusetts General Hospital have even undergone training for the pioneering surgery. But no one knows when a suitable patient will arrive through the doors. That is in fact one of the issues they face: by the nature of trauma, patients won’t be able to give informed consent for the procedure. Because of this, Tisherman’s group has engaged in a wide community consultation to let citizens in the area know the programme was going on. The study had to be signed off personally by the Secretary of the Army, the highest-ranking civilian official in the organisation.
Beyond that lie further obstacles. Amid the frantic activity of the emergency room, Tisherman must make sure that a team of trauma surgeons can work in concert with cardiac surgeons and perfusionists armed with pumps and bags of chilled saline, an additional layer of complexity in an already chaotic environment. And although cooling affects all tissues equally, it is not without secondary effects. The blood factors responsible for clotting are also inhibited by the cold. This creates problems controlling bleeding during the rewarming phase. The surgeons, too, will suffer from the cold, as both the patient and the room itself will be chilled during the procedure. Yet the cold is only a tool; the end goal is metabolic suspension.
In the future, emergency preservation and resuscitation could be extended to those suffering heart attacks or exposure to poisons, or any critical care situation where time is a factor. “Cooling is the most powerful way of suppressing metabolism we have,” says Tisherman, “If we can either decrease the needs of the tissues or improve oxygen delivery to the tissues then everything will be okay.”
Although animals in the laboratory were able to recover from three hours in this suspended state, the first human patients to experience it will only be put under for a third of that. “An hour should be enough to repair the bleeding,” Tisherman says. “The cooling period doesn’t necessarily have to cover the entire surgery.” For those wanting to travel to distant stars, going beyond that hour is, sadly, out of the question for now. “We’re not trying to freeze the dead,” Tisherman chuckles, “just buy enough time to save the living.”
This article was fist appeared in Mosaic, which is dedicated to exploring the science of life, and reproduced under a Creative Commons licence. For more Mosaic articles click here.
If you would like to comment on this, or anything else you have seen on Future, head over to our Facebook or Google+ page, or message us on Twitter.
'예전포스팅' 카테고리의 다른 글
동영상음원 추출하기 (0) | 2015.04.10 |
---|---|
How often do you need to see a dentist? (0) | 2015.04.10 |
수면장애를 겪고 계세요? (0) | 2015.04.09 |
수면 관련 좌담회 (0) | 2015.04.09 |
Sleep: How to nap like a pro (0) | 2015.04.08 |